In the long run, we will implement evaporation from the water blocks, but for now we concentrate upon the heat exchange between the surface and the atmosphere. In previous versions of our program, we allowed sunlight to pass directly into the surface gas cells. Now we allow sunlight to pass all the way through the entire atmosphere to be absorbed at a solid or liquid surface. Real water reflects 4% of short-wave radiation, and sand reflects something like 10%, but our simulated water and sand absorbs all short-wave radiation.
Both sand and water are near-perfect emitters of long-wave radiation, so we allow our surface blocks to radiate heat according to Stefan's Law. We also allow heat to leave the surface blocks by convection. We determine the convection heat loss by multiplying the temperature difference between the surface and the gas by a convection coefficient. This heat leaves the surface block and enters the gas cell above.
As we described in our previous post, our simulated atmosphere is partially-transparent, to an extent specified by the transparency fraction, τ. The gas above a surface block absorbs a fraction 1−τ of the block's radiation, and the remainder passes out into space. We add the absorbed heat to the gas cell above the surface block.
Now we come to a component in the heat exchange between the atmosphere and the surface that we have not simulated or calculated before. The atmosphere itself will radiate heat downwards towards the surface, and the surface, being a perfect absorber of such radiation, will absorb all of it. The heat radiated downward by the atmosphere is often called back-radiation or downward long-wave radiation. To calculate the back-radiation, we use the same equation we applied to the tropopause in our previous post. When the transparency fraction is 0.5, the gas radiates half as much heat as a black body at the same temperature.
We ran our simulation with Day heating of 350 W/m2, all surface blocks made of sand, convection coefficient 20 W/m2K, and transparency fraction 0.50. The following figure shows the equilibrium state of the array. The surface blocks are color-coded for temperature in the same way as the gas cells, but they have an orange border to mark them as sand. A water cell has a blue border. You will find the equilibrium state of the array saved as a text file here.
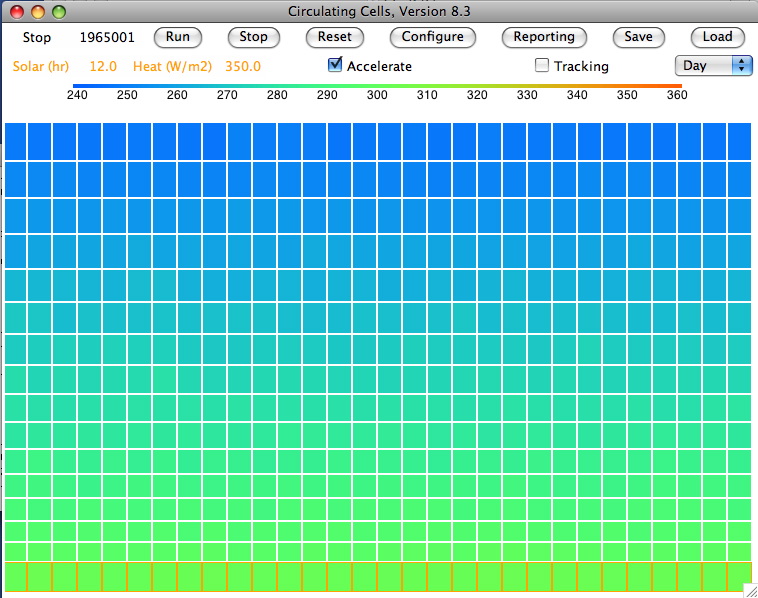
As in our previous simulations, the temperature drop from the surface gas cells to the tropopause gas cells is very close to 50 K. The sandy surface settles to 303.0 K, at which temperature it radiates 480 W/m2. Of this, 240 W/m2 passes directly into space and 240 W/m2 is absorbed by the gas above. The tropopause settles to 249.0 K and radiates 110 W/m2 into space. The total radiation into space is 350 W/m2, which is the amount that is arriving from the sun, so our planet is in thermal equilibrium.
The 110 W/m2 radiated by the tropopause must pass up through the atmosphere by convection. The surface gas is at an average temperature of 298.5 K, radiating 226 W/m2 back to the surface. Thus a net 14 W/m2 passes from the surface to the atmosphere by radiation. The remaining 96 W/m2 passes into the atmosphere by convection at the surface. The surface is on average 4.5 K warmer than the gas, for which we expect only 90 W/m2 to flow by convection. But the gas cells warm by roughly 8 K while they sit upon the sand. When they first arrive from above, they are almost 10 K cooler, and 200 W/m2 flows into them by convection. Just before they rise up, they are only 2 K cooler, at which point only 40 W/m2 flows into them. When we get the program to print out the convection rate, we obtain an average of 96 W/m2, so all the heat from the Sun is accounted for.
We note that almost all the heat flowing from the surface to the atmosphere is carried by convection. The heat radiated by the surface and absorbed by the atmosphere is nearly balanced by the radiation returned by this same atmosphere. The difference is only 14 W/m2, compared to 96 W/m2 passing into the gas by convection. In our next post, we will simulate night and day over a sandy desert and see if we come up with reasonable variations in temperature at the sandy surface, the air above the surface, and the air high up in the tropopause.
No comments:
Post a Comment